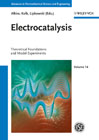
Volume XIV in the series Advances in Electrochemical Sciences and Engineering provides a valuable overview of this rapidly developing field by focusing on the aspects that drive the research of today and tomorrow. As such it covers the fundamentals as well as an overview of the most important applications, showing how the latest methods are used in the field, including current spectroscopic methods and computational approaches Top scientists from different areas, both theoretical and experimental, share their expertise on this hot topic, making it a must–have for many different disciplines, including chemistry, physics, biochemistry, engineering as well as surface and materials science. INDICE: Preface XIII List of Contributors XV 1 Multiscale Modeling of Electrochemical Systems 1 Jonathan E. Mueller, Donato Fantauzzi, and Timo Jacob 1.1 Introduction 1 1.2 Introduction to Multiscale Modeling 3 1.3 Electronic Structure Modeling 6 1.3.1 Modern Electronic Structure Theory 6 1.3.1.1 Quantum Mechanical Foundations 6 1.3.1.2 Born–Oppenheimer Approximation 8 1.3.1.3 Single–Electron Hamiltonians 9 1.3.1.4 Basis Sets 10 1.3.1.5 Enforcing the Pauli Principle 11 1.3.1.6 Electron Correlation Methods 12 1.3.1.7 Density Functional Theory 14 1.3.2 Applications of Electronic Structure to Geometric Properties 16 1.3.2.1 Geometry Optimization 16 1.3.2.2 Transition State Searches 17 1.3.3 Corrections to Potential Energy Surfaces and Reaction Pathways 18 1.3.3.1 Energy and Entropy Corrections 18 1.3.3.2 Thermodynamic State Functions 20 1.3.3.3 Reaction Energies and Rates 21 1.3.4 Electronic Structure Models in Electrochemistry 22 1.3.4.1 Modeling the Electrode Surface: Cluster versus Slab 23 1.3.4.2 Modeling the Solvent: Explicit versus Implicit 24 1.3.4.3 Modeling the Electrode Potential 25 1.3.5 Summary 26 1.4 Molecular Simulations 27 1.4.1 Energy Terms and Force Field Parameters 27 1.4.1.1 Covalent Bond Interactions 27 1.4.1.2 Non–Covalent Interactions 32 1.4.2 Parametrization and Validation 34 1.4.3 Atomistic Simulations 35 1.4.3.1 Monte Carlo Methods 35 1.4.3.2 Molecular Dynamics 36 1.4.3.3 QM/MM 37 1.4.4 Sampling and Analysis 39 1.4.5 Applications of Molecular Modeling in Electrochemistry 39 1.4.6 Summary 40 1.5 Reaction Modeling 40 1.5.1 Introduction 40 1.5.2 Chemical Kinetics 41 1.5.3 Kinetic Monte Carlo 41 1.5.3.1 System States and the Lattice Approximation 41 1.5.3.2 Reaction Rates 42 1.5.3.3 Reaction Dynamics 43 1.5.3.4 Applications of kMC in Electrochemistry 45 1.5.4 Summary 45 1.6 The Oxygen Reduction Reaction on Pt(111) 46 1.6.1 Introduction to the Oxygen Reduction Reaction 46 1.6.2 Preliminary Considerations 46 1.6.3 DFT Calculations 48 1.6.4 Method Validation 49 1.6.5 Reaction Energies 49 1.6.6 Solvation Effects 51 1.6.7 Free Energy Contributions 52 1.6.8 Influence of an Electrode Potential 53 1.6.9 Modeling the Kinetic Rates 55 1.6.10 Summary 58 1.7 Formic Acid Oxidation on Pt(111) 59 1.7.1 Introduction to Formic Acid Oxidation 59 1.7.2 Density Functional Theory Calculations 60 1.7.3 Gas Phase Reactions 60 1.7.4 Explicit Solvation Model 61 1.7.5 Eley–Rideal Mechanisms and the Electrode Potential 63 1.7.6 Kinetic Rate Model of Formic Acid Oxidation 65 1.7.7 Summary 66 1.8 Concluding Remarks 66 References 67 2 Statistical Mechanics and Kinetic Modeling of Electrochemical Reactions on Single–Crystal Electrodes Using the Lattice–Gas Approximation 75 Marc T.M. Koper 2.1 Introduction 75 2.2 Lattice–Gas Modeling of Electrochemical Surface Reactions 76 2.3 Statistical Mechanics and Approximations 79 2.3.1 Static System 79 2.3.2 Dynamical System 83 2.4 Monte Carlo Simulations 84 2.5 Applications to Electrosorption, Electrodeposition and Electrocatalysis 85 2.5.1 Electrosorption and Electrodeposition 85 2.5.2 Electrocatalysis 92 2.6 Conclusions 96 References 96 3 Single Molecular Electrochemistry within an STM 99 Richard J. Nichols and Simon J. Higgins 3.1 Introduction 99 3.2 Experimental Methods for Single Molecule Electrical Measurements in Electrochemical Environments 101 3.3 Electron Transfer Mechanisms 103 3.3.1 Tunneling 106 3.3.2 Resonant Tunneling 109 3.3.3 Hopping Models 111 3.4 Single Molecule Electrochemical Studies with an STM 115 3.4.1 Adsorbed Iron Complexes 115 3.4.2 Viologens 118 3.4.3 Osmium and Cobalt Metal Complexes 122 3.4.4 PyrroloTTF (pTTF) 125 3.4.5 Perylene Tetracarboxylic Diimides 128 3.4.6 Oligo(phenylene ethynylene) Derivates 130 3.5 Conclusions and Outlook 131 References 134 4 From Microbial Bioelectrocatalysis to Microbial Bioelectrochemical Systems 137 Uwe Schr€oder and Falk Harnisch 4.1 Prelude: From Fundamentals to Biotechnology 137 4.2 Microbial Bioelectrochemical Systems (BESs) 137 4.2.1 The Archetype: Microbial Fuel Cells (MFCs) 137 4.2.2 Strength Through Diversity: Microbial Bioelectrochemical Systems 139 4.3 Bioelectrocatalysis: Microorganisms Catalyze Electrochemical Reactions 140 4.3.1 Energetic Considerations of Microbial Bioelectrocatalysis 141 4.3.1.1 Case Study: The Anodic Acetate Oxidation by Geobacteraceae 142 4.3.1.2 Case Study: The Cathodic Hydrogen Evolution Reaction (HER) 143 4.3.2 Microbial Electron Transfer Mechanisms 144 4.3.2.1 Direct Electron Transfer (DET) 144 4.3.2.2 Mediated Electron Transfer (MET) 146 4.3.2.3 Cathodic Electron Transfer Mechanisms 148 4.3.3 Microbial Interactions: Ecological Networks 148 4.3.3.1 Interspecies Electron Transfer and “Scavenging” of Redox–Shuttles 148 4.4 Characterizing Anodic Biofilms by Electrochemical and Biological Means 149 4.4.1.1 Case Study: On the use of Cyclic Voltammetry 154 4.4.1.2 Case Study: Raman Microscopy 155 References 156 5 Electrocapillarity of Solids and its Impact on Heterogeneous Catalysis 163 J€org Weissm€uller 5.1 Introduction 163 5.2 Mechanics of Solid Electrodes 164 5.2.1 Outline – Surface Stress and Surface Tension 164 5.2.2 Solid Versus Fluid 167 5.2.3 Free Energy of Elastic Solid Surfaces 167 5.2.4 Deforming a Solid Surface 170 5.2.5 Case Study: Thought Experiment in Electrowetting 172 5.2.6 Capillary Equations for Fluids and Solids 175 5.2.7 Case Study: Molecular Dynamics Study of Surface–Induced Pressure 177 5.3 Electrocapillary Coupling at Equilibrium 177 5.3.1 Outline – Polarizable and Nonpolarizable Electrodes 177 5.3.2 Lippmann Equation and Electrocapillary Coupling Coefficient 179 5.3.3 Case Study: Cantilever–Bending Experiment in Electrolyte 181 5.3.4 Important Maxwell Relations for Electrocapillarity 183 5.3.5 Electrocapillary Coupling During Electrosorption 184 5.3.6 Coupling Coefficient for Adsorption from Gas 185 5.3.7 Coupling Coefficient for the Langmuir Isotherm 186 5.3.8 Case Study: Strain–Dependent Hydrogen Underpotential Deposition 187 5.3.9 Coupling Coefficient for Potential of Zero Charge and Work Function 190 5.3.10 Empirical Data for the Electrocapillary Coupling Coefficient 193 5.4 Exploring the Dynamics 198 5.4.1 Outline 198 5.4.2 Cyclic Cantilever–Bending Experiments 199 5.4.3 Dynamic Electro–Chemo–Mechanical Analysis 200 5.5 Mechanically Modulated Catalysis 203 5.5.1 Outline 203 5.5.2 Phenomenology; Distinguishing Capacitive from Faraday Current 204 5.5.3 Rate equations: Butler–Volmer kinetics 206 5.5.4 Rate Equations: Heyrowsky Reaction 207 5.6 Summary and Outlook 212 References 215 6 Synthesis of Precious Metal Nanoparticles with High Surface Energy and High Electrocatalytic Activity 221 Long Huang, Zhi–You Zhou, Na Tian, and Shi–Gang Sun 6.1 Introduction 221 6.2 Shape–Controlled Synthesis of Monometallic Nanocrystals with High Surface Energy 224 6.2.1 Electrochemical Route 224 6.2.1.1 Platinum 224 6.2.1.2 Palladium 228 6.2.2 Wet–Chemical Route 230 6.2.2.1 Platinum 230 6.2.2.2 Palladium 232 6.2.2.3 Gold 234 6.3 Shape–Controlled Synthesis of Bimetallic NCs with High Surface Energy 235 6.3.1 Surface Modification 236 6.3.1.1 Bi–Modified THH Pt NCs 237 6.3.1.2 Ru–Modified THH Pt NCs 239 6.3.1.3 Au–Modified Pt THH NCs 241 6.3.1.4 Pt–Modified Au Prisms with High–Index Facets 243 6.3.2 Alloy NCs 245 6.3.2.1 THH PdPt Alloy 245 6.3.2.2 HOH PdAu Alloy 247 6.3.3 Core–Shell Structured NCs 248 6.4 Concluding Remarks and Perspective 254 References 256 7 X–Ray Studies of Strained Catalytic Dealloyed Pt Surfaces 259 Peter Strasser 7.1 Introduction 259 7.2 Dealloyed Bimetallic Surfaces 262 7.3 Dealloyed Strained Pt Core–Shell Model Surfaces 264 7.4 X–Ray Studies of Dealloyed Strained PtCu3(111) Single Crystal Surfaces 266 7.5 X–Ray Studies of Dealloyed Strained Pt–Cu Polycrystalline Thin Film Surfaces 269 7.6 X–Ray Studies of Dealloyed Strained Alloy Nanoparticles 273 7.6.1 Bragg Brentano Powder X–Ray Diffraction (XRD) 273 7.6.2 In Situ High Temperature Powder X–Ray Diffraction (XRD) 274 7.6.3 Synchrotron X–Ray Photoemission Spectroscopy (XPS) 276 7.6.4 Anomalous Small Angle X–Ray Scattering (ASAXS) 277 7.6.5 Anomalous Powder X–Ray Diffraction (AXRD) 277 7.6.6 High Energy X–Ray Diffraction (HE–XRD) and Atomic Pair Distribution Function (PDF) Analysis 281 7.7 Conclusions 284 References 285 Index 293
- ISBN: 978-3-527-33227-4
- Editorial: Wiley VCH
- Encuadernacion: Cartoné
- Páginas: 320
- Fecha Publicación: 18/12/2013
- Nº Volúmenes: 1
- Idioma: Inglés