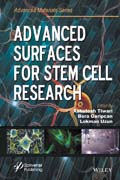
Advanced Surfaces for Stem Cell Research
Tiwari, Ashutosh
Garipcan, Bora
Uzun, Lokman
INDICE: Preface xv .1 Extracellular Matrix Proteins for Stem Cell Fate 1 Betül Çelebi–Saltik .1.1 Human Stem Cells, Sources, and Niches 2 .1.2 Role of Extrinsic and Intrinsic Factors 5 .1.2.1 Shape 5 .1.2.2 Topography Regulates Cell Fate 6 .1.2.3 Stiffness and Stress 6 .1.2.4 Integrins 7 .1.2.5 Signaling via Integrins 9 .1.3 Extracellular Matrix of the Mesenchyme: Human Bone Marrow 11 .1.4 Biomimetic Peptides as Extracellular Matrix Proteins 13 .References 15 .2 The Superficial Mechanical and Physical Properties of Matrix Microenvironment as Stem Cell Fate Regulator 23 Mohsen Shahrousvand, Gity Mir Mohamad Sadeghi and Ali Salimi .2.1 Introduction 24 .2.2 Fabrication of the Microenvironments with Different Properties in Surfaces 25 .2.3 Effects of Surface Topography on Stem Cell Behaviors 28 .2.4 Role of Substrate Stiffness and Elasticity of Matrix on Cell Culture 31 .2.5 Stem Cell Fate Induced by Matrix Stiffness and Its Mechanism 32 .2.6 Competition/Compliance between Matrix Stiffness and Other Signals and Their Effect on Stem Cells Fate 33 .2.7 Effects of Matrix Stiffness on Stem Cells in Two Dimensions versus Three Dimensions 34 .2.8 Effects of External Mechanical Cues on Stem Cell Fate from Surface Interactions Perspective 34 .2.9 Conclusions 35 .Acknowledgments 36 .References 36 .3 Effects of Mechanotransduction on Stem Cell Behavior 43 Bahar Bilgen and Sedat Odabas .3.1 Introduction 43 .3.2 The Concept of Mechanotransduction 45 .3.3 The Mechanical Cues of Cell Differentiation and Tissue Formation on the Basis of Mechanotransduction 46 .3.4 Mechanotransduction via External Forces 47 .3.4.1 Mechanotransduction via Bioreactors 48 .3.4.2 Mechanotransduction via Particle–based Systems 51 .3.4.3 Mechanotransduction via Other External Forces 53 .3.5 Mechanotransduction via Bioinspired Materials 54 .3.6 Future Remarks and Conclusion 54 .Declaration of Interest 55 .References 55 .4 Modulation of Stem Cells Behavior Through Bioactive Surfaces 65 Eduardo D. Gomes, Rita C. Assunção–Silva, Nuno Sousax, Nuno A. Silva and António J. Salgado .4.1 Lithography 66 .4.2 Micro and Nanopatterning 70 .4.3 Microfluidics 71 .4.4 Electrospinning 71 .4.5 Bottom–up/Top–down Approaches 74 .4.6 Substrates Chemical Modifications 75 .4.6.1 Biomolecules Coatings 76 .4.6.2 Peptide Grafting 77 .4.7 Conclusion 78 .References 79 .Contents vii .5 Influence of Controlled Micro– and Nanoengineered Environments on Stem Cell Fate 85 Anna Lagunas, David Caballero and Josep Samitier .5.1 Introduction to Engineered Environments for the Control of Stem Cell Differentiation 86 .5.1.1 Stem Cells Niche In Vivo: A Highly Dynamic and Complex Environment 86 .5.1.2 Mimicking the Stem Cells Niche In Vitro: Engineered Biomaterials 88 .5.2 Mechanoregulation of Stem Cell Fate 89 .5.2.1 From In Vivo to In Vitro: Influence of the Mechanical Environment on Stem Cell Fate 89 .5.2.2 Regulation of Stem Cell Fate by Surface Roughness 90 .5.2.3 Control of Stem Cell Differentiation by Micro– and Nanotopographic Surfaces 92 .5.2.4 Physical Gradients for Regulating Stem Cell Fate 96 .5.3 Controlled Surface Immobilization of Biochemical Stimuli for Stem Cell Differentiation 100 .5.3.1 Micro– and Nanopatterned Surfaces: Effect of Geometrical Constraint and Ligand Presentation at the Nanoscale 100 .5.3.2 Biochemical Gradients for Stem Cell Differentiation 107 .5.4 Three–dimensional Micro– and Nanoengineered Environments for Stem Cell Differentiation 112 .5.4.1 Three–dimensional Mechanoregulation of Stem Cell Fate 113 .5.4.2 Three–dimensional Biochemical Patterns for Stem Cell Differentiation 119 .5.5 Conclusions and Future Perspectives 122 .References 122 .6 Recent Advances in Nanostructured Polymeric Surface: Challenges and Frontiers in Stem Cells 141 Ilaria Armentano, Samantha Mattioli, Francesco Morena, Chiara Argentati, Sabata Martino, Luigi Torre and Josè Maria Kenny .6.1 Introduction 142 .6.2 Nanostructured Surface 144 .6.3 Stem Cell 146 .6.4 Stem Cell/Surface Interaction 147 .6.5 Microscopic Techniques Used in Estimating Stem Cell/Surface 148 .6.5.1 Fluorescence Microscopy 148 .6.5.2 Electron Microscopy 149 .6.5.3 Atomic Force Microscopy 153 .6.5.3.1 Instrument 154 .6.5.3.2 Cell Nanomechanical Motion 156 .6.5.3.3 Mechanical Properties 156 .6.6 Conclusions and Future Perspectives 158 .References 158 .7 Laser Surface Modification Techniques and Stem Cells Applications 165 Ça r Kaan Akkan .7.1 Introduction 166 .7.2 Fundamental Laser Optics for Surface Structuring 166 .7.2.1 Definitive Facts for Laser Surface Structuring 167 .7.2.1.1 Absorptivity and Reflectivity of the Laser Beam by the Material Surface 167 .7.2.1.2 Effect of the Incoming Laser Light Polarization 168 .7.2.1.3 Operation Mode of the Laser 169 .7.2.1.4 Beam Quality Factor 170 .7.2.1.5 Laser Pulse Energy/Power 171 .7.2.2 Ablation by Laser Pulses 172 .7.2.2.1 Focusing the Laser Beam 172 .7.2.2.2 Ablation Regime 173 .7.3 Methods for Laser Surface Structuring 174 .7.3.1 Physical Surface Modifications by Lasers 174 .7.3.1.1 Direct Structuring 175 .7.3.1.2 Beam Shaping Optics 177 .7.3.1.3 Direct Laser Interference Patterning 180 .7.3.2 Chemical Surface Modification by Lasers 181 .7.3.2.1 Pulsed Laser Deposition 181 .7.3.2.2 Laser Surface Alloying 184 .7.3.2.3 Laser Surface Oxidation and Nitriding 186 .7.4 Stem Cells and Laser–Modified Surfaces 187 .7.5 Conclusions 191 .References 192 .8 Plasma Polymer Deposition: A Versatile Tool for Stem Cell Research 197 M. N. Macgregor–Ramiasa and K. Vasilev .8.1 Introduction 197 .8.2 The Principle and Physics of Plasma Methods for Surface Modification 199 .8.2.1 Plasma Sputtering, Etching an Implantation 200 .8.2.2 Plasma Polymer Deposition 201 .8.3 Surface Properties Influencing Stem Cell Fate 202 .8.3.1 Plasma Methods for Tailored Surface Chemistry 203 .8.3.1.1 Oxygen–rich Surfaces 204 .8.3.1.2 Nitrogen–rich Surfaces 208 .8.3.1.3 Systematic Studies and Copolymers 210 .8.3.2 Plasma for Surface Topography 211 .8.3.3 Plasma for Surface Stiffness 213 .8.3.4 Plasma for Gradient Substrata 215 .8.3.5 Plasma and 3D Scaffolds 218 .8.4 New Trends and Outlook 219 .8.5 Conclusions 219 .References 220 .9 Three–dimensional Printing Approaches for the Treatment of Critical–sized Bone Defects 231 Sara Salehi, Bilal A. Naved and Warren L. Grayson .9.1 Background 232 .9.1.1 Treatment Approaches for Critical–sized Bone Defects 232 .9.1.2 History of the Application of 3D Printing to Medicine and Biology 233 .9.2 Overview of 3D Printing Technologies 234 .9.2.1 Laser–based Technologies 235 .9.2.1.1 Stereolithography 235 .9.2.1.2 Selective Laser Sintering 236 .9.2.1.3 Selective Laser Melting 236 .9.2.1.4 Electron Beam Melting 237 .9.2.1.5 Two–photon Polymerization 237 .9.2.2 Extrusion–based Technologies 238 .9.2.2.1 Fused Deposition Modeling 238 .9.2.2.2 Material Jetting 238 .9.2.3 Ink–based Technologies 239 .9.2.3.1 Inkjet 3D Printing 239 .9.2.3.2 Aerosol Jet Printing 239 .9.3 Surgical Guides and Models for Bone Reconstruction 240 .9.3.1 Laser–based Surgical Guides 240 .9.3.2 Extrusion–based Surgical Guides 240 .9.3.3 Ink–based Surgical Guides 241 .9.4 Three–dimensionally Printed Implants for Bone Substitution 242 .9.4.1 Laser–based Technologies for Metallic Bone Implants 244 .9.4.2 Extrusion–based Technologies for Bone Implants 245 .9.4.3 Ink–based Technologies for Bone Implants 246 .9.5 Scaffolds for Bone Regeneration 246 .9.5.1 Laser–based Printing for Regenerative Scaffolds 247 .9.5.2 Extrusion–based Printing for Regenerative Scaffolds 247 .9.5.3 Ink–based Printing for Regenerative Scaffolds 249 .9.5.4 Pre– and Postprocessing Techniques 250 .9.5.4.1 Preprocessing 250 .9.5.4.2 Postprocessing: Sintering 256 .9.5.4.3 Postprocessing: Functionalization 256 .9.6 Bioprinting 257 .9.7 Conclusion 262 .List of Abbreviation 263 .References 264 .10 Application of Bioreactor Concept and Modeling Techniques to Bone Regeneration and Augmentation Treatments 277 Oscar A. Deccó and Jésica I. Zuchuat .10.1 Bone Tissue Regeneration 278 .10.1.1 Proinflammatory Cytokines 279 .10.1.2 Transforming Growth Factor Beta 279 .10.1.3 Angiogenesis in Regeneration 280 .10.2 Actual Therapeutic Strategies and Concepts to .Obtain an Optimal Bone Quality and Quantity 281 .10.2.1 Guided Bone Regeneration Based on Cells 282 .10.2.1.1 Embryonic Stem Cells 282 .10.2.1.2 Adult Stem Cells 282 .10.2.1.3 Mesenchymal Stem Cells 283 .10.2.2 Guided Bone Regeneration Based on PRP and Growth Factors 284 .10.2.2.1 Bone Morphogenetic Proteins 287 .10.2.3 Guided Bone Regeneration Based on Barrier Membranes 288 .10.2.4 Guided Bone Regeneration Based on Scaffolds 290 .10.3 Bioreactors Employed for Tissue Engineering in Guided Bone Regeneration 291 .10.4 Bioreactor Concept in Guided Bone Regeneration and Tissue Engineering: In Vivo Application 294 .10.5 New Multidisciplinary Approaches Intended to Improve and Accelerate the Treatment of Injured and/or Diseased Bone 303 .10.5.1 Application of Bioreactor in Dentistry: Therapies for the Treatment of Maxillary Bone Defects 304 .10.5.2 Application of Bioreactor in Cases of Osteoporosis 307 .10.6 Computational Modeling: An Effective Tool to Predict Bone Ingrowth 310 .References 311 .11 Stem Cell–based Medicinal Products: Regulatory Perspectives 321 DenizOzdil and Halil Murat Aydin .11.1 Introduction 321 .11.2 Defining Stem Cell–based Medicinal Products 323 .11.3 Regional Regulatory Issues for Stem Cell Products 326 .11.4 Regulatory Systems for Stem Cell–based Technologies 327 .11.4.1 The US Regulatory System 328 .11.5 Stem Cell Technologies: The European .Regulatory System 336 .References 340 .12 Substrates and Surfaces for Control of Pluripotent Stem Cell Fate and Function 341 Akshaya Srinivasan, Yi–Chin Toh, Xian Jun Loh and Wei Seong Toh .12.1 Introduction 342 .12.2 Pluripotent Stem Cells 342 .12.3 Substrates for Maintenance of Self–renewal and Pluripotency of PSCs 344 .12.3.1 Cellular Substrates 344 .12.3.2 Acellular Substrates 345 .12.3.2.1 Biological Matrices 345 .12.3.2.2 ECM Components 348 .12.3.2.3 Decellularized Matrices 350 .12.3.2.4 Cell Adhesion Molecules 351 .12.3.2.5 Synthetic Substrates 352 .12.4 Substrates for Promoting Differentiation of PSCs 355 .12.4.1 Cellular Substrates 355 .12.4.2 Acellular Substrates 356 .12.4.2.1 Biological Matrices 356 .12.4.2.2 ECM Components 358 .12.4.2.3 Decellularized Matrices 362 .12.4.2.4 Cell Adhesion Molecules 363 .12.4.2.5 Synthetic Substrates 363 .12.5 Conclusions 366 .Acknowledgments 367 .References 367 .13 Silk as a Natural Biopolymer for Tissue Engineering 379 Ay e Ak Can and Gamze Bölükba i Ate .13.1 Introduction 380 .13.2 SF as a Biomaterial 383 .13.2.1 Fibroin Hydrogels and Sponges 384 .13.2.2 Fibroin Films and Membranes 386 .13.2.3 Nonwoven and Woven Silk Scaffolds 386 .13.2.4 Silk Fibroin as a Bioactive Molecule Delivery 386 .13.3 Biomedical Applications of Silk–based Biomaterials 387 .13.3.1 Bone Tissue Engineering 387 .13.3.2 Cartilage Tissue Engineering 389 .13.3.3 Ligament and Tendon Tissue Engineering 391 .13.3.4 Cardiovascular Tissue Engineering 391 .13.3.5 Skin Tissue Engineering 393 .13.3.6 Other Applications of Silk Fibroin 393 .13.4 Conclusion and Future Directions 393 .References 394 .14 Applications of Biopolymer–based, Surface–modified Devices in Transplant Medicine and Tissue Engineering 399 Ashim Malhotra, Gulnaz Javan and Shivani Soni .14.1 Introduction to Cardiovascular Disease 400 .14.2 Need Assessment for Biopolymer–based Devices in Cardiovascular Therapeutics 400 .14.3 Emergence of Surface Modification Applications in Cardiovascular Sciences: A Historical Perspective 401 .14.4 Nitric Oxide Producing Biosurface Modification 403 .14.5 Surface Modification by Extracellular Matrix Protein Adherence 404 .14.6 The Role of Surface Modification in the Construction of Cardiac Prostheses 405 .14.7 Biopolymer–based Surface Modification of Materials Used in Bone Reconstruction 406 .14.8 The Use of Biopolymers in Nanotechnology 409 .14.8.1 Protein Nanoparticles 410 .14.8.1.1 Albumin–based Nanoparticles and Surface Modification 411 .14.8.1.2 Collagen–based Nanoparticles and Surface Modification 412 .14.8.1.3 Gelatin–based Nanoparticle Systems 413 .14.8.2 Polysaccharide–based Nanoparticle Systems 413 .14.8.2.1 The Use of Alginate for Surface Modifications 413 .14.8.2.2 The Use of Chitosan–based Nanoparticles and Chitosan–based Surface Modification 414 .14.8.2.3 The Use of Chitin–based Nanoparticles and Chitin–based Surface Modification 416 .14.8.2.4 The Use of Cellulose–based Nanoparticles and Cellulose–based Surface Modification 417 .References 418 .15 Stem Cell Behavior on Microenvironment Mimicked Surfaces 423 M. Özgen Öztürk Öncel and Bora Garipcan .15.1 Introduction 424 .15.2 Stem Cells 425 .15.2.1 Definition and Types 425 .15.2.1.1 Embryonic Stem Cells 426 .15.2.1.2 Adult Stem Cells 426 .15.2.1.3 Reprogramming and Induced Pluripotent Stem Cells 427 .15.2.2 Stem Cell Niche 427 .15.3 Stem Cells: Microenvironment Interactions 428 .15.3.1 Extracellular Matrix 429 .15.3.2 Signaling Factors 429 .15.3.3 Physicochemical Composition 430 .15.3.4 Mechanical Properties 430 .15.3.5 Cell Cell Interactions 431 .15.4 Biomaterials as Stem Cell Microenvironments 431 .15.4.1 Surface Chemistry 431 .15.4.2 Surface Hydrophilicity and Hydrophobicity 434 .15.4.3 Substrate Stiffness 435 .15.4.4 Surface Topography 435 .15.5 Biomimicked and Bioinspired Approaches 436 .15.5.1 Bone Tissue Regeneration 439 .15.5.2 Cartilage Tissue Regeneration 440 .15.5.3 Cardiac Tissue Regeneration 441 .15.6 Conclusion 442 .References 442
- ISBN: 978-1-119-24250-5
- Editorial: John Wiley & Sons
- Encuadernacion: Cartoné
- Páginas: 470
- Fecha Publicación: 23/12/2016
- Nº Volúmenes: 1
- Idioma: Inglés